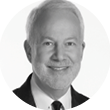
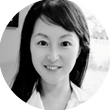
Considerations of optimal pupil size used to be a largely intellectual exercise. Today, the subject has practical implications given the number of pupil-modulating drops and small-aperture IOLs in development to treat presbyopia. This new approach to presbyopia was heralded by the recent first-in-class FDA approval of Vuity (pilocarpine HCl ophthalmic solution 1.25%, Allergan) and affirmative decision on the premarket approval application for the IC-8 small-aperture IOL (AcuFocus), both for the treatment of presbyopia.
Changes in pupillary diameter are highly dynamic; they are influenced by accommodative effort, lighting, refractive error, emotional state, age, and other factors. Psychophysical studies suggest that, for a well-focused eye, pupil size iterates to whatever achieves the best visual acuity,1 grating detection,2 and retinal image quality3 for viewing objects at various levels of environmental light.
Studies of visual performance when the entrance pupil is artificially modified by viewing through small apertures speak directly to the original query: What is the optimal pupil size? As this article explains, the answer to this question is that it depends. The pupillary diameter to optimize distance, intermediate, or near vision can differ because it is affected by a number of determining factors, including retinal illuminance, diffraction, lower-order aberrations (LOAs; ie, defocus), and higher-order aberrations (HOAs).
This article attempts to provide a framework that will help eye care practitioners to understand pupillary modulation more fully and evaluate the benefits and compromises involved in pupillary modulation during a person’s typical day.
UNDERLYING FACTORS
For a well-focused eye (defocus = 0 D), the optical impact of pupillary diameter is modulated by the competing negative effects of diffraction with smaller pupils and the blur generated by optical aberrations with larger pupils. In eyes with no HOAs, retinal image quality increases proportionately to pupillary diameter owing to increases in the diffraction bandwidth. In aberrated eyes, however, contrast decreases with pupillary dilation because of the impact of aberrations on the retinal point spread function (Figure 1). For normal, well-focused eyes with an average level of HOAs, this interplay generally results in an optimum photopic pupil size of somewhere between 2 and 3 mm vis-à-vis visual acuity and retinal image quality.1-3 This optimal pupil size can be even smaller for highly aberrated eyes (eg, those with keratoconus or a history of radial keratotomy or LASIK).4
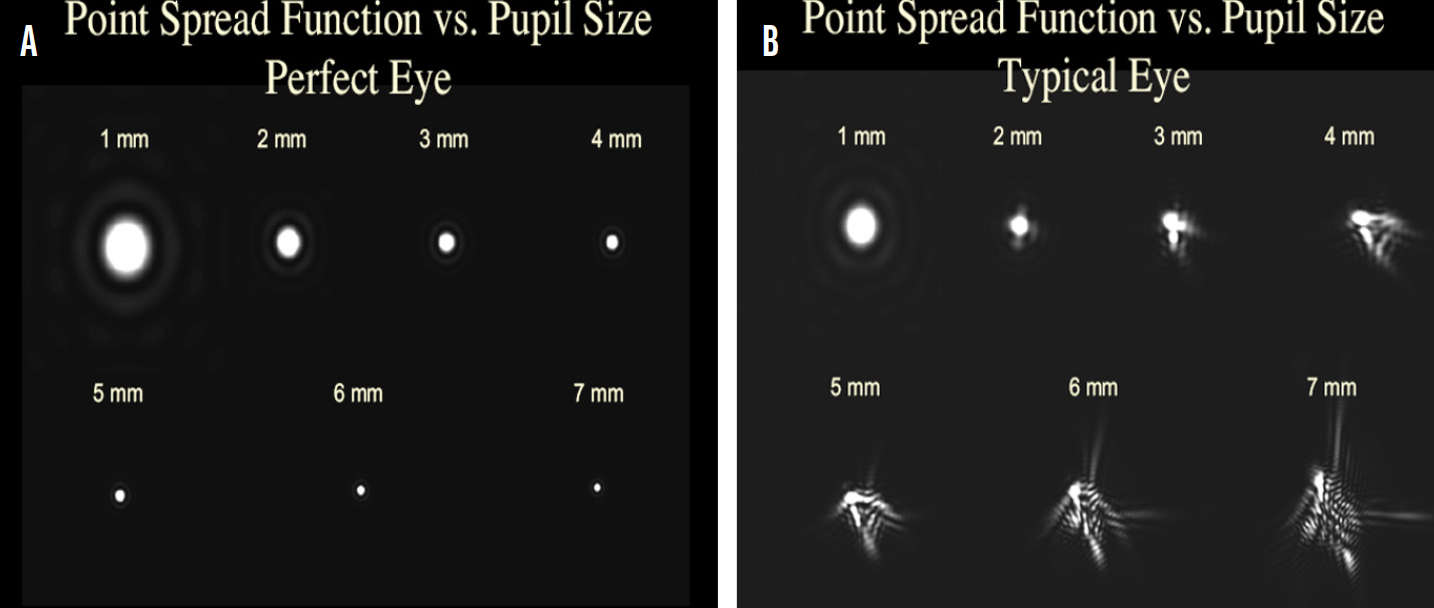
Figure 1. In an eye with no HOAs (A), the quality of the retinal point spread image worsens with smaller pupils owing to the negative impact of diffraction. In an eye with HOAs (B), retinal image quality degrades with larger pupils owing to the negative impact of HOAs on the retinal point spread function.
At photopic light levels, the decrease in retinal illuminance produced by constricting the pupil with strong miotics has no effect on vision because the retinal neural contrast threshold changes proportionately to background illumination (a relationship known as Weber’s law).5 Under dim illumination and with significant miosis, however, if retinal illuminance declines below 100 trolands (a troland is a measure of the angular flux density of light incident upon the retina6), quantal fluctuations change the tradeoff between diffraction and aberrations because photon noise greatly elevates the retinal neural contrast threshold. At lower light levels, this change in the signal-to-noise ratio due to photon noise is known as the deVries-Rose law, in which the change in retinal image quality is the square root of change in retinal illuminance. Consequently, under mesopic conditions the ideal pupil size increases to about 6 mm. In dim light conditions, the detrimental effects of aberrations are outweighed by the dramatic decline in retinal contrast sensitivity.4,7
The aforementioned factors apply to a well-focused eye. Presbyopic eyes, however, constantly experience varying levels of defocus when viewing objects at near or intermediate distance. In presbyopic eyes, a small pupil reduces the retinal blur size according to the following equation: retinal blur size in degrees visual angle = 0.057 x defocus in diopters x pupillary diameter in millimeters.8 Miosis narrows the conoid of Sturm by blocking skewed peripheral light rays. Narrowing the pupil will proportionally reduce the retinal blur circle, thereby diminishing the impact of defocus on retinal image quality and expanding depth of focus.8
Interestingly, pupillary miosis has a different impact on myopic defocus than on hyperopic defocus. As seen in Figure 2, broadening the defocus curve is of greater advantage in patients who are slightly myopic than in those who are emmetropic or somewhat hyperopic. This is because hyperopic individuals cannot clinically appreciate the impact of flattening and broadening the left side of the defocus curve (plus diopter defocus). Those with low myopia, however, receive the positive benefit of both sides of the flatter, broader defocus curve—dramatically enhanced depth of field. This effect can be even greater for people with early presbyopia who retain some residual accommodative ability, which can be combined with the enhanced pseudoaccomodating effect of miosis.
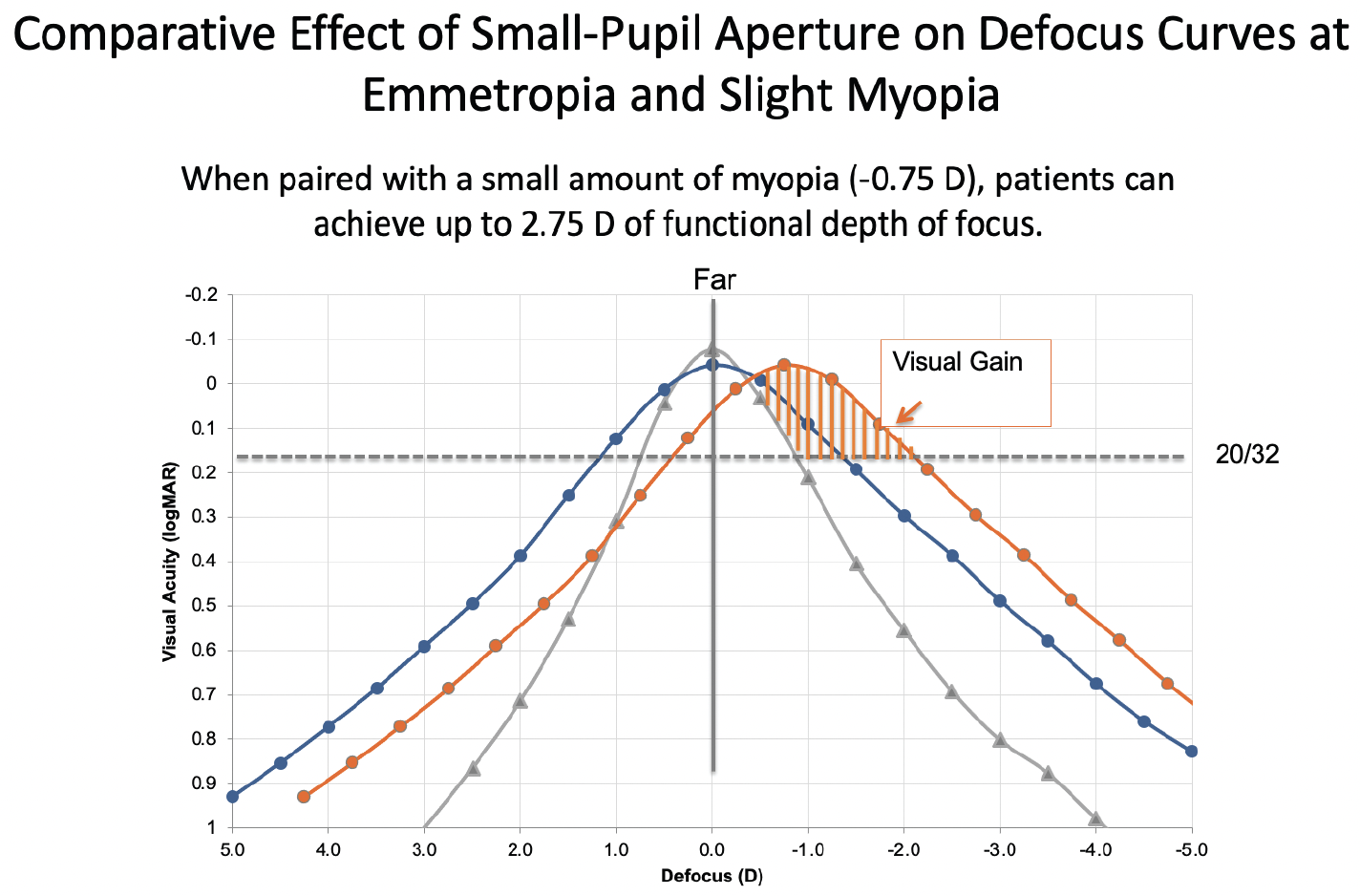
Figure 2. The monocular photopic defocus curve (gray) of a patient with a 3-mm pupil and a monofocal IOL. Note the broader monocular defocus curve (blue) in a patient who received an IC-8 IOL with a 1.36-mm aperture targeted for emmetropia. The functional depth of field further expands (orange) when a patient with an IC-8 IOL is targeted for -0.75 D, whereas the impact on uncorrected distance visual acuity is minimal because the patient now benefits from widening of both sides of the defocus curve.
In short, the impact of pupillary modulation on functional depth of field differs among patients who are slightly hyperopic or myopic versus those who are truly emmetropic.
HOAS AND SCATTER
In general, older eyes that are more highly aberrated have a smaller optimum pupil size because the benefit in image quality from reduced HOAs may offset the photon noise effect more, even in dim light. In addition to the impact of HOAs, which deflect light over small angles, ocular forward scatter causes the uniform distribution of light across a wide region of the retina and subsequently reduces the modulation transfer function.9 Ocular forward scatter thus must also be taken into account.
For example, a patient with keratoconus and an inferior cone with resultant high coma may have a smaller optimum pupil size even at night than a young patient with few HOAs, whereas a patient with early nuclear sclerosis may be more significantly affected by a miotic pupil at night because the light absorbance of the human crystalline lens increases exponentially as it ages10 and the resulting optics (aberrations plus forward light scattering) associated with central nuclear sclerosis are poor.
POTENTIAL CONSEQUENCES IF THE ENTRANCE PUPIL IS TOO SMALL
When retinal illuminance is low, small pupillary diameters degrade image quality via diffraction, reduce contrast sensitivity, and obviate the beneficial effect of decreased optical aberrations, as illustrated in the following case. A patient with a highly aberrated eye and traumatic mydriasis achieved good vision after receiving an XtraFocus Pinhole Implant (Morcher) with a 1.3-mm aperture (Figure 3A) but asked to have the IOL explanted because of bare perception of objects at night in dim light.11
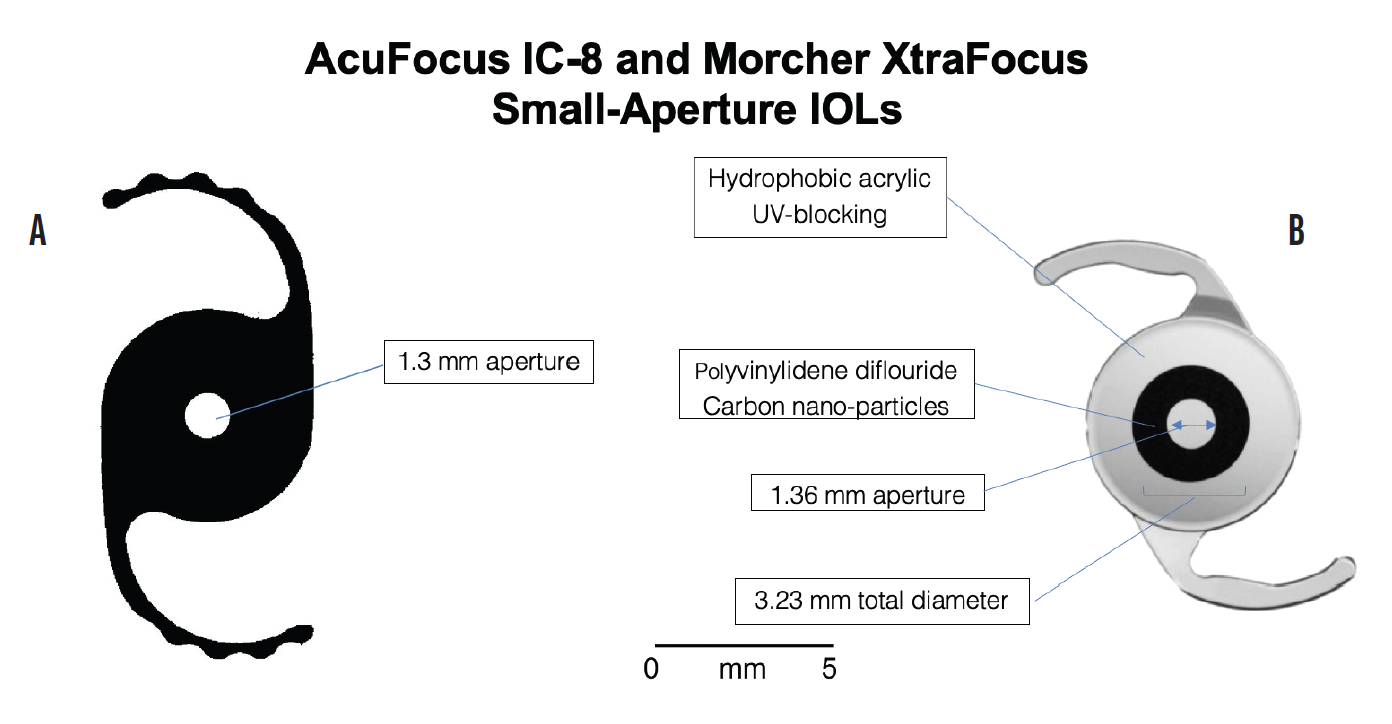
Figure 3. The Morcher XtraFocus Pinhole IOL (A) is a sulcus-fixated, piggyback IOL made of foldable, black hydrophobic material that is completely transparent to infrared light, allowing viewing of posterior structures using infrared equipment such as OCT, scanning laser ophthalmoscopy, or an infrared slit lamp. The IC-8 is an acrylic, hydrophobic small-aperture IOL (B). The embedded annular mask has an outer diameter of 3.23 mm with a central aperture measuring 1.36 mm in diameter. The mask contains 3,200 microperforations on its annulus (sparing the periphery) ranging in size from 7 to 10 μm in diameter and arranged in a pseudorandom fashion.
Studies have suggested that some light transilluminates through the iris and sclera of patients with lightly versus darkly pigmented eyes, altering the more fixed relationship between pupillary diameter and retinal illumination in patients with brown irides.12 Strong miotic drops or an opaque nonannular pinhole IOL may have a more negative impact on distance vision in low light, possibly to a greater extent in people with dark irides. This effect is more likely to be revealed by mesopic contrast sensitivity or low-contrast mesopic vision tests and could be missed by less sensitive modes of evaluation. The use of a miotic drop in only one eye has been proposed to mitigate the negative impact of low retinal illumination on contrast sensitivity. This approach, however, foregoes the benefit of binocular summation at near, and it introduces the possibility that some patients will experience the Pulfrich phenomenon (ie, distortions in the perception of relative motion) owing to interocular differences in visual latency.13
The aforementioned case, in which a small-aperture IOL was explanted because of poor night vision, illustrates the potential benefit of maintaining some degree of pupil reactivity (different pupillary diameters under mesopic vs photopic conditions), which may be retained with certain miotic drops or drop combinations but lost with others. In other words, there may be a tradeoff between the duration of action of miotic drops, the degree of miosis, and the maintenance of some pupillary response. A dark-eyed person who is happy with improved near vision in the afternoon after the administration of a strong miotic drop could find driving home in the dark at the end of the workday challenging because of reduced retinal illumination and retinal contrast sensitivity from photon noise lowering. This also has implications for the development of IOLs with differently sized central apertures that can be mixed and matched to accentuate depth of field without causing difficulties under mesopic or scotopic conditions where retinal illumination is reduced.
Another case in point is a patient with irregular corneal astigmatism and traumatic mydriasis who achieved excellent UCVA and depth of field and a reduction in glare and photophobia after receiving an opaque XtraFocus Pinhole Implant.14 This individual requested explantation of the IOL because it greatly accentuated his awareness of significant floaters. If floaters reside predominantly in the path of the visual axis, isolating these rays of light with a small pupil may have a negative impact.
Unlike reports of retinal contrast issues11 or floaters14 related to small-aperture, opaque XtraFocus IOLs, reports of glare and halos in patients with an IC-8 microperforated small-aperture annulus IOL (AcuFocus) are related to a larger pupil size, with more severe symptoms reported by individuals whose mesopic pupils are larger than 5.6 mm.15 Younger patients with irregular corneal astigmatism or scarring who receive an IC-8 may be more likely to experience these unwanted side effects because their natural pupil size under mesopic light16 typically exceeds the 5.6-mm threshold. The use of miotic drops under development, however, may mitigate these photic phenomena. This solution may be an alternative to manufacturing annulus IOLs with masks of varying diameters for this subset of patients.
A concern about using pinhole optics as a therapeutic treatment modality is their potential to reduce the visual field. This has certainly been the case when spectacles17 or contact lenses18,19 have been used to create a small pupil on the spectacle or corneal plane (rather than the ideal pupillary plane), and it has been observed to a small degree in patients with a Kamra inlay (CorneaGen).20,21 Small-aperture IOLs located close to the pupillary plane22 and pharmacological pupillary miosis will not reduce the visual field or cause scotomas as a direct result of geometric optics. A global reduction in visual field, however, has been reported in patients who received 2% pilocarpine, especially when pupillary diameter was less than 2 mm,23,24 because of reduced retinal illumination due to the photon noise effect. Unlike the true vignetting effect observed in eyes with a small aperture (ie, Kamra inlay), the reduction of visual field associated with pharmacological miosis (< 2 mm) can be reversed simply by increasing the light level. It will be important to determine whether strong miotics that reduce pupillary diameter to below 2 mm (eg, aceclidine or a fixed combination of carbochol and brimonidine) similarly result in visual field changes under standard testing conditions—changes that may be exacerbated and become clinically apparent under mesopic or scotopic light levels.
CONCLUSION
Optimal pupil size and modulation depend on a delicate balance among the image quality of objects at varying degrees of defocus, LOAs, HOAs, ocular forward light scatter, and retinal contrast sensitivity—the last profoundly affected by photon noise in dim light. Under varying lighting conditions and retinal illumination, the optimal pupil size for viewing objects at distance, intermediate, and near often differs, and optimization of one may be to the detriment of another. It is possible that the degree of iris and uveal pigmentation also play a role by affecting retinal illumination under different lighting conditions, adding a confounding variable.
Nonfenestrated small-aperture IOLs that are diffusely opaque from the aperture to the edge of the optic will reduce retinal illumination more than the IC-8, which has a 1.36-mm aperture and a microperforated annulus of 3.23 mm outer diameter with a surrounding clear zone (Figure 3B). Drops that create a fixed, nonreactive pupil for a long duration may improve vision at near, intermediate, and distance under photopic conditions but could markedly reduce retinal contrast thresholds under dim light, which could affect individuals’ ability to drive at night or work under varying lighting conditions. People are generally unlikely to experience uniform lighting throughout the course of a day. It is worth noting that commercially available pilocarpine drops carry a warning to exercise caution when driving at night and pursuing hazardous occupations in poor illumination.
Different miotic agents under development for the treatment of presbyopia appear to have varying durations of action and effects on pupillary diameter and reactivity.25 Most work as cholinergic agonists, triggering the iris sphincter, whereas phentolamine mesylate 1% (Nyxol, Ocuphire Pharma) is a nonselective, competitive, reversible alpha adrenergic antagonist that directly inhibits the iris dilator muscle (Figure 4). Appropriate dosing of selective single-agent miotics or the use of combination, nonfixed-dose miotics (eg, phentolamine mesylate 1% plus low-dose pilocarpine) may offer a more nuanced ability to modulate the pupil. There will be inherent tradeoffs, however, in image quality under different lighting conditions and depth of field that will be influenced by an individual’s inherent LOAs, HOAs, light scatter, and other factors.
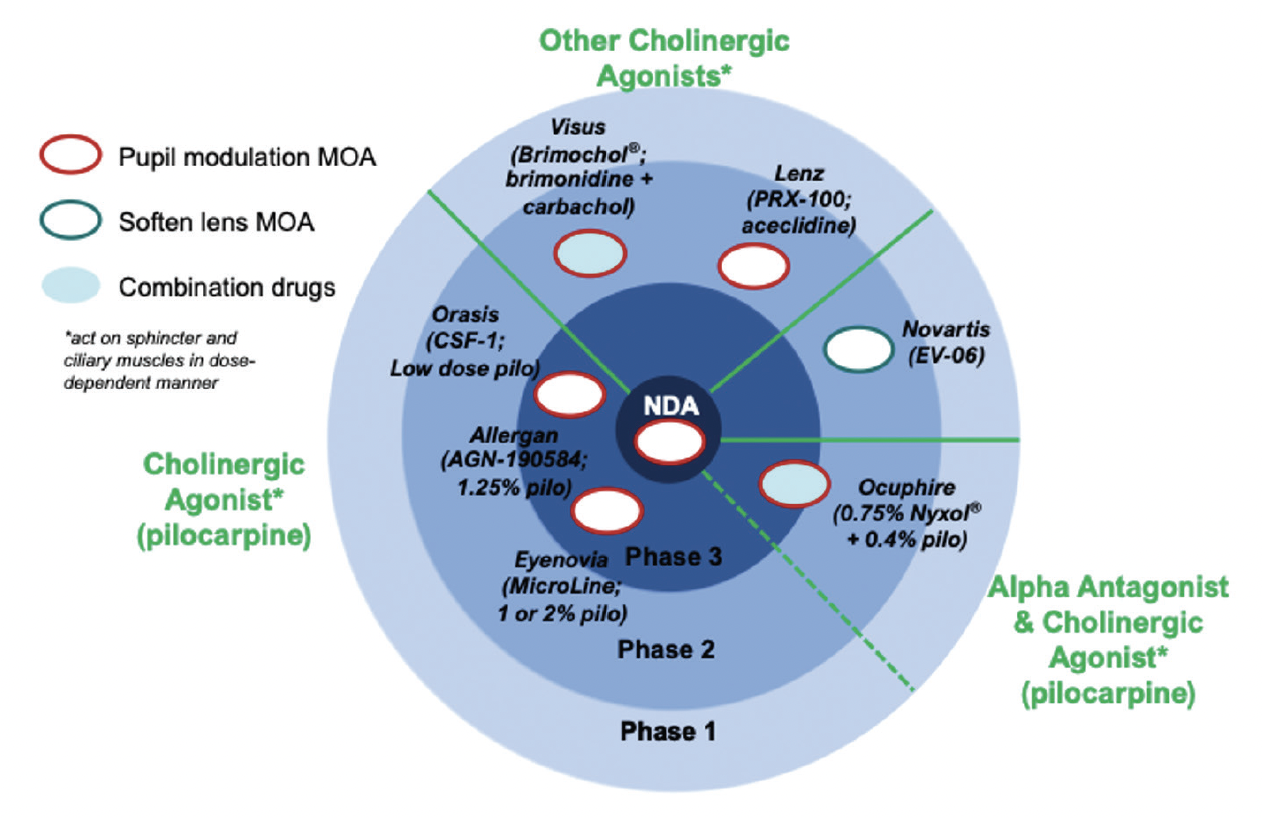
Figure 4. The developmental landscape for presbyopia-treating drops segmented by proposed mechanism of action (MOA) and stage of development vis-à-vis FDA-registered studies.
Abbreviation: NDA, new drug application
Image created based on information from manufacturers’ websites and Grzybowski A, Markeviciute A, Zemaitiene R. A review of pharmacological presbyopia treatment. Asia Pac J Ophthalmol (Phila). 2020;9(3):226-233.
One size is unlikely to fit all. Because presbyopia is a common physiological ocular malady, there should be ample opportunity to use a number of fixed-dose and combination nonfixed-dose miotic drugs and small-aperture IOL offerings to customize treatment and thereby maximize the benefits for each patient.
1. Campbell FW, Gregory AH. Effect of size of pupil on visual acuity. Nature. 1960;187:1121-1123.
2. Woodhouse JM. The effect of pupil size on grating detection at various contrast levels. Vision Res. 1975;15:645-648.
3. Xu R, Thibos L, Bradley A. Effect of target luminance on optimum pupil diameter for presbyopic eyes. Optom Vis Sci. 2016;93:1409-1419.
4. Xu R, Wang H, Thibos LN, Bradley A. Interaction of aberrations, diffraction, and quantal fluctuations determine the impact of pupil size on visual quality. J Opt Soc Am A Opt Image Sci Vis. 2017;34:481-492.
5. Van Nes FL, Bouman MA. Spatial modulation transfer in the human eye. Journal of the Optical Society of America. 1967;57(3):401-406.
6. Thibos LN, Lopez-Gil N, Bradley A. What is a troland? J Opt Soc Am A Opt Image Sci Vis. 2018;35(5):813-816.
7. Kollbaum PS, Bradley A. Correction of presbyopia: old problems with old (and new) solutions. Clin Exp Optom. 2020;103:21-30.
8. Smith G. Angular diameter of defocus blur discs. Am J Optom Physiol Opt. 1982;59:885-889.
9. van Oterendorp C, Diaz-Santana L, Bull N, et al. Light scattering and wavefront aberrations in in vivo imaging of the rat eye: a comparison study. Invest Ophthalmol Vis Sci. 2011;52:4551-4559.
10. Weale RA. Age and the transmittance of the human crystalline lens. J Physiol. 1988;395:577-587.
11. Agarwal P, Navon SE, Subudhi P, Mithal N. Persistently poor vision in dim illumination after implantation of Xtrafocus small-aperture IOL (Morcher). BMJ Case Rep. 2019;12.
12. Kardon RH, Hong S, Kawasaki A. Entrance pupil size predicts retinal illumination in darkly pigmented eyes, but not lightly pigmented eyes. Invest Ophthalmol Vis Sci. 2013;54:5559-5567.
13. Plainis S, Petratou D, Giannakopoulou T, Radhakrishnan H, Pallikaris IG, Charman WN. Interocular differences in visual latency induced by reduced-aperture monovision. Ophthalmic Physiol Opt. 2013;33(2):123-129.
14. Agarwal P, Navon SE. Persistent troublesome floaters necessitating the explantation of XtraFocus Pinhole IOL (Morcher). BMJ Case Rep. 2019;12:e229057.
15. Dick HB, Piovella M, Vukich J, Vilupuru S, Lin L; Clinical Investigators. Prospective multicenter trial of a small-aperture intraocular lens in cataract surgery. J Cataract Refract Surg. 2017;43(7):956-968.
16. Winn B, Whitaker D, Elliott DB, Phillips NJ. Factors affecting light-adapted pupil size in normal human subjects. Invest Ophthalmol Vis Sci. 1994;35(3):1132-1137.
17. Hallett JW, Pittler S. Individually made acrylic moist-chamber spectacles and pinhole glasses. Am J Ophthalmol. 1946;29:725-728.
18. Freeman E. Pinhole contact lenses. Am J Optom Arch Am Acad Optom. 1952;29:347-352.
19. Insler MS, Hendricks C, George DM. Visual field constriction caused by colored contact lenses. Arch Ophthalmol. 1988;106(12):1680-1682.
20. Langenbucher A, Goebels S, Szentmary N, et al. Vignetting and field of view with the Kamra corneal inlay. Biomed Res Int. 2013;2013:154593.
21. Vukich JA, Durrie DS, Pepose JS, Thompson V, van de Pol C, Lin L. Evaluation of the small-aperture intracorneal inlay: three-year results from the cohort of the U.S. Food and Drug Administration clinical trial. J Cataract Refract Surg. 2018;44:541-556.
22. Srinivasan S, Wei Khoo L, Koshy Z. Posterior segment visualization in eyes with small-aperture intraocular lens. J Refract Surg. 2019;35(8):538-542.
23. McCluskey DJ, Douglas JP, O’Connor PS, Story K, Ivy LM, Harvey JS. The effect of pilocarpine on the visual field in normals. Ophthalmology. 1986;93:843-846.
24. Lindenmuth KA, Skuta GL, Rabbani R, Musch DC. Effects of pupillary constriction on automated perimetry in normal eyes. Ophthalmology. 1989;96:1298-1301.
25. Katz JA, Karpecki PM, Dorca A, et al. Presbyopia – a review of current treatment options and emerging therapies. Clin Ophthalmol. 2021;15:2167-2178.