Variations in shape and corneal biomechanics among patients have the greatest impact on the predictability of anterior segment surgical outcomes. Improving predictability and reducing outliers would be highly valuable for treatments such as astigmatic relaxing incisions and intrastromal corneal ring segment (ICRS) implantation and for identifying the risk of post-LASIK ectasia.
AT A GLANCE
- IOP and stresses in corneal tissue form a biomechanical balance, thereby defining the shape of each individual patient’s cornea.
- A validation study confirmed that finite-element surgery simulation is capable of closely predicting the corneal component of cataract surgical outcomes.
- Personalization becomes more important as educated patients—especially in the premium market—ask for optimal surgical outcomes.
- Predictive modeling can be used to customize surgical parameters for any given patient specifically, and may, ultimately, be important in daily clinical practices for risk assessment, surgical planning, and outcomes prediction.
IOP and stresses in corneal tissue form a biomechanical balance, thereby defining the shape of each individual patient’s cornea. Pathologies such as keratoconus and surgical treatments such as LASIK, cataract removal, arcuate incision creation, ICRS implantation, and CXL modify the delicate balance and—whether desired or undesired—may substantially change the shape and consequently the refractive behavior of the patient’s cornea.
State-of-the-art surgical planning is mainly based on an individual surgeon’s experience and on his or her statistical information, such as a personalized nomogram. Although long-term experience will always be key to the success of any treatment, application of nomograms too often fails to catch outliers. Because today’s laser devices provide a high level of precision, we propose that it is rather patients’ unique biomechanical characteristics that contribute to the outliers.
If this is true, patient-specific surgical planning—based on accurate and reliable biomechanical simulation technology—has great potential to reduce outliers, improve safety, and decrease medical costs as a result of lower retreatment rates. The ultimate question is this: Will it ever be possible to provide robust, patient-specific predictive planning for anterior segment surgery?
OPHTHALMO-BIOMECHANICAL MODELING AND SIMULATION
In order to answer the above question, three things must be established:
1. an accurate mathematical description of corneal biomechanical properties,
2. the means to perform surgery simulation and outcome prediction, and
3. robust clinical validation once the predictive simulation tool-chain is established.
Description of biomechanical properties. Although other modeling methods exist, including mass-spring and finite difference, the most comprehensive approach to simulating the biomechanics of soft biologic tissue is the finite element method. Thereby, the specimen’s geometry is reproduced by a volume mesh of elements of finite size. In combination with nonlinear continuum mechanics, the finite element method allows the modeling of material nonlinearity, incompressibility, inhomogeneity, and anisotropy. The material properties are formulated as a so-called strain-energy function:

On the left side of the equation, (Ψ) is the energy needed to deform the material; the right side expresses deformation. In particular, V is tissue compression (change in volume), and ΨM is deformation in the nonfibril tissue matrix. Collagen fiber stretching is expressed by ΨF, along with the weighting function Φ, which defines the amount of collagen fibers for each corneal meridian (1 – 360°). ΨxL is the collagen crosslinks (or bifurcated fibers) connecting main fibers and adding shear-stiffness to the material definition. The amount of ΨxL is dependent on the position within the corneal thickness profile, where we find more bifurcated fibers anteriorly than posteriorly.1
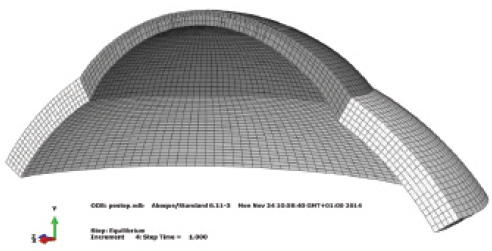
Figure 1. Finite-element model of the cornea (gray) and parts of the sclera (white). The brick-like elements are hexahedral finite elements that form the 3-D volume mesh. IOP is simulated on the inside of the model. The model was cut in half for display; simulations are carried out with full 3-D models.
The energy (force) response of the law in this equation was calibrated using cadaver mechanical inflation and strip extensiometry experiments in corneal tissue.2 By using dissected corneas from cadaver eyes of different ages, the law can be further fine-tuned to match patient age.
Surgery simulation. The approach to finite-element surgery simulation is the same for various treatments. An example uses a template finite-element volume mesh of an average spherical cornea with an anterior radius of curvature of 7.72 mm, a central corneal thickness of 545 µm, and a posterior radius of curvature of 6.34 mm. Furthermore, a scleral band of 4 mm width is attached to the cornea model in the limbus. The sclera is modeled with 1 mm thickness and an outer radius of 12 mm (Figure 1).
This template is subsequently warped to patient data acquired by corneal topography or anterior segment OCT devices, such as the Pentacam (Oculus Optikgeräte) and Galilei (Ziemer Ophthalmic Systems). These patient-specific finite element volume meshes can be used to compute the initial stress distribution physiologically caused by the patient’s IOP.
The third step in the simulation is treatment-specific, where, for example, virtual arcuate keratotomy (AK) incisions are cut, virtual LASIK flaps opened, or virtual inlays implanted into corneal models (Figure 2). In a last step, clinically relevant information such as curvatures, pachymetry, elevation, or wavefront aberration can be computed based on the surfaces of the simulation models (Figure 3).
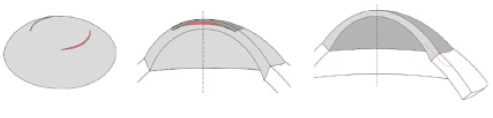
Figure 2. Examples of finite-element models: Simulations of arcuate keratotomy, with the incisions in red (A), of an implant inserted into the cornea, with the implant in red (B), and of a cataract phaco incision, with the incision in red (C). Wherever the model is cut in half, this is for visualization purposes. Full 3-D models are used in all simulations.
Validation. The simulation tool explained above—from patient-specific finite-element 3-D volume mesh generation, through surgery simulation with estimated biomechanical properties of the cornea, to the calculation of surface information based on the simulation models—is implemented and available for use in the Optimeyes software (Integrated Scientific Services). It was validated in a prospective, observational clinical study at the University Hospital in Zurich, Switzerland, in which surgically induced astigmatism after phaco surgery was investigated.3
Preoperative Pentacam measurements were used to simulate cataract treatment in 13 patients. The simulation outcome was then compared with follow-up Pentacam measurements of the same eyes taken at more than 1-month postoperative. Results showed that predicted astigmatic change was reasonably close to the clinically observed astigmatic change. The prediction error was -0.07 ±0.19 D of sphere, and -0.01 ±0.38 D of cylinder. This validation study supports the suggestion that the proposed methodology is capable of closely predicting the corneal component of cataract surgical outcome. Figure 4 shows case examples from the study, comparing simulation predictions versus clinical postoperative measurements.
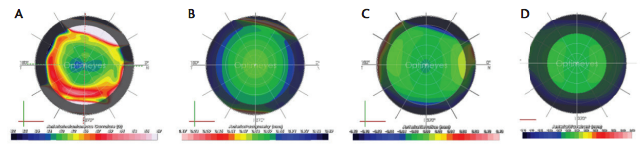
Figure 3. Examples of information calculated on a post-LASIK model cornea: Instantaneous curvature (A), pachymetry (B), best-fit-sphere elevation (C), and wavefront aberration (D). Shadowed areas on the maps indicate extrapolated information.
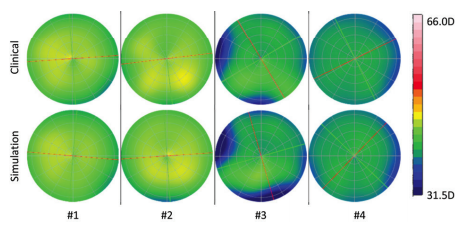
Figure 4. Cataract validation study: These four examples, comparing simulation outcomes to clinical postoperative outcomes, show that the predictions were close to the clinically measured data. Depicted are axial curvatures, color-coded with the Galilei’s color scale.
Similar but as yet unpublished material on LASIK, cataract surgery, CXL in keratoconus, corneal implant surgery, and femtosecond intrastromal relaxing incision surgery indicates the validity of outcomes prediction by biomechanical simulations of various types of surgical interventions. These data support the general applicability of the methods described above to anterior segment eye surgery. Publication of this material is planned.
APPLICATIONS AND DISCUSSION
There are many potential clinical and industrial applications for biomechanical simulations in anterior segment eye surgery, whether for planning, prediction, or optimization. Applications in industry typically involve the development of implantable products or corneal incision architecture as well as parametric studies of established or new surgical treatments to identify the sensitivity of individual surgical parameters (Figure 5).
In line with the general trend toward personalized medicine, application of biomechanical simulations in daily clinical practice could include the customization and personalization of corneal surgical treatments for individual patients. Personalization becomes more important as educated patients—especially in the premium market—ask for optimal surgical outcomes.
Biomechanical simulation software, either online or embedded in surgical laser or diagnostic devices, could predict such things as the optimal parameters for intrastromal relaxing incisions, in order to compensate for a particular patient’s astigmatism, or could define optimal ICRS placement for keratoconus surgery. In LASIK, biomechanical simulations could be important for preoperative estimation of ectasia risk and might also predict unexpected individual biomechanical deformations in topography-guided LASIK and other personalized ablation patterns, such as Supracor presbyopia treatments with the Technolas 217P laser (Bausch + Lomb Technolas).
CONCLUSION
As asserted more than a decade ago, the cornea is not a piece of plastic,4 and study of its distinct biomechanical behavior relative to corneal surgical outcomes remains a growing field of interest and research. This research continues to unveil the impact that corneal biomechanics has on anterior segment treatments. The delicate mechanical balance between IOP and corneal stresses, when altered, leads to modifications of shape and function, and therefore affects most corneal treatments in some way.
Patient-specific biomechanical simulations, in combination with software that calculates shape and functionally related information on the surfaces of postsimulation models, will allow prediction of outcomes of anterior segment surgeries. Such simulations can be employed in so-called optimization loops, in which surgical parameters are optimized in order to achieve predefined goals that may include minimizing corneal astigmatism, for example. Hence, with optimization loops, predictive modeling can be used to customize surgical parameters for any given patient specifically and can, ultimately, be important in our daily clinical practices for risk assessment, surgical planning, and outcomes prediction.

Figure 5. Example applications in which biomechanical simulations have been used in research and design projects in the industry. Simulations were applied to questions relating to corneal incisions (A), corneal implants (B), LASIK and ReLEx small incision lenticule extraction (C), and contact lens applications (D).
In order to further improve patient-specificity of biomechanical simulations, it will be important to include potential local variations of corneal mechanical properties. Such information is not currently measurable in vivo because it would require destruction of the tissue. Technologies that might ultimately provide reliable assessment of patient-specific biomechanical properties include Brillouin microscopy and shear wave elastography. Although devices such as the Ocular Response Analyzer (Reichert Technologies) and the Corvis ST (Oculus Optikgeräte) provide clinically relevant biomechanical information, the values they provide cannot yet be directly plugged into biomechanical simulations. Research with these devices continues.
Although there is room for improvement, predictive modeling with patient-specific corneal shape, IOP, and age-matched mechanical properties is already capable of closely reproducing clinical results and of predicting outcomes for various types of surgical treatments. Therefore, addressing the question from the beginning of this article, we can say this: Yes, it is possible to provide patient-specific planning assistance in ophthalmic surgery.
1. Petsche SJ, Chemyak D, Martiz J, et al. Depth-dependent transverse shear properties of the human corneal stroma. Invest Ophthalmol Vis Sci. 2012;52(2):873-880.
2. Studer HP. Biomechanical model of human cornea based on stromal microstructure. J Biomech. 2010;43(5):836-842.
3. Studer HP. Patient-specific finite-element simulation of the human cornea: A clinical validation study on cataract surgery. J Biomech. 2013;46(4):751-758.
4. Roberts CJ. The cornea is not a piece of plastic. J Refract Surg. 2000;16:407-413.
Cynthia J. Roberts, PhD
– Professor of Ophthalmology and Biomedical Engineering, Department of Ophthalmology and Department of Biomedical Engineering, The Ohio State University, Columbus, Ohio
– roberts.8@osu.edu
– Financial disclosure: Consultant (Ziemer Ophthalmic Systems, Oculus Optikgeräte); Advisory Board (Optimeyes); Research/travel funding (Carl Zeiss Meditec, Euclid Systems)
Harald P. Studer, PhD
– Senior Software and Biomedical Engineer, Integrated Scientific Services, Biel, Switzerland
– harald.studer@iss-ag.ch
– Financial disclosure: Financial interest (Optimeyes software)