From the first utilization of the excimer laser for photoablative corneal reshaping to the emergence of the femtosecond laser for flap creation and later for intrastromal lenticular extraction, laser refractive surgery has matured into a safe and predictable method of surgical vision correction. Based on our clinical experience in therapeutic laser refractive surgery, this article emphasizes five potentially important issues that may often be overlooked without noticeable consequences when treating virgin eyes. These issues can prove to be crucial, however, in the treatment of complex irregular corneas—as in post-LASIK decentrations, in keratoconus, or in eyes after corneal injuries or keratitis.
Fundamental 1: EPITHELIAL CONTRIBUTION TO CORNEAL OPTICS
In techniques in which the epithelium is removed before laser ablation—such as traditional PRK, epi-LASIK, and LASEK—all preoperative measurements on which the treatments are based are performed with the epithelium intact. However, the refractive treatment itself is executed on the stromal surface after epithelial removal. The predictability of outcomes in such treatments is based on the assumption that the epithelium does not significantly contribute to the total corneal optics. However, this does not seem to be the case.
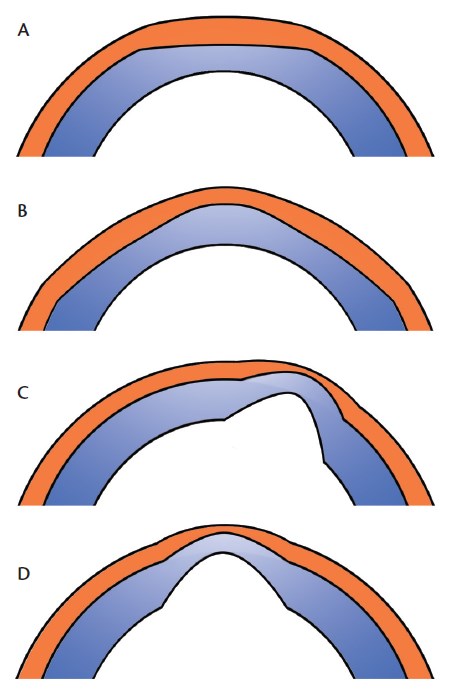
Figure 1. Schematic drawing of cross-sectional corneal images showing epithelial remodeling after myopic treatment (A), after hyperopic treatment (B), in keratoconus (C), and in corneal ectasia secondary to myopic treatment (D).
Simon et al1 found that the epithelium accounted for an average 1.03 D higher central optical power in deepithelialized eyes compared with eyes that had an intact epithelium. The corneal epithelium in normal virgin eyes is thinnest in the center and gradually increases in thickness toward the periphery,2 contributing negative refractive power. However, even if the epithelium were of even thickness, a deepithelialized cornea would have a smaller radius—that is, a steeper curvature—than it did before epithelial removal, as removal of a concentric layer from a sphere causes a decrease in radius.1
Additionally, corneal epithelium is an active layer that compensates for change in the stromal surface's shape by getting thinner over the bumps or islands and thicker over the divots and relative depressions. This phenomenon is called epithelial remodeling (Figure 1).3 An increase in central epithelial thickness to fill the depressed center of the corneal stroma after myopic LASIK4-7 and PRK7,8 has been shown to increase the anterior corneal curvature and reduce the effect of surgery.
Epithelial remodeling has an even greater effect on the optical properties of eyes with irregular stromas. In eyes with irregular astigmatism9 and keratoconus,10,11 the epithelium acts as a mask that evens out the underlying stromal irregularities to achieve a smoother and more optically symmetric surface.
To treat remaining irregularities that are beyond the reach of epithelial remodeling, topography-guided ablation can be used. In such cases, surface ablation is normally used instead of LASIK. However, in order to facilitate the smoothing effect of epithelial remodeling, surface ablation must be applied transepithelially or, alternatively, only if preceded by a refractive-neutral transepithelial PTK to a depth matching the maximum depth of the epithelium. This will remove the epithelium and the protruding stromal bumps underneath, achieving a shape mirroring that of the corneal surface. This would also be the only way to perform a topography-guided ablation on a surface that matches the topography taken during the preoperative examination on which the ablation plan is based.
Two high-resolution corneal imaging technologies have been reported to provide the necessary precision for mapping the corneal epithelium: anterior segment OCT12 and Artemis very–high-frequency digital ultrasound (Ultralink).13
The refractive contribution of the epithelium is of most concern in eyes with irregular astigmatism and in retreatments of previous refractive surgery; however, it must be accounted for to improve the predictability of the outcomes in any type of surface ablation, particularly refractive surgery enhancements, in which the epithelial refractive component may make a big difference in predictability.
Fundamental 2: CONTRIBUTION OF HOAs TO MANIFEST REFRACTION
Manifest refraction is used as a gold standard in refractive surgery planning and in prescribing visual aids. The examination is performed as a process in which the patient is systematically presented with combinations of spherical and cylindrical lenses in search for the one that forms the retinal image with least diffusion and distortion. All optical aberrations decrease the quality of retinal images; higher-order aberrations (HOAs) cause visual distortions and decrease visual acuity even in the absence of spherocylindrical error.14-16 Unfortunately, subjective refraction limited to spherical and cylindrical correction can neither detect the amount of HOAs in an eye nor measure the contribution of various HOAs to the spherocylindrical manifest refraction. Based on a computational model, it has been calculated that a root-mean-square (RMS) HOA of 0.43 mm or greater is equivalent to at least 0.50 D of spherical error for a 5-mm pupil aperture.17 For some clinical scenarios, see the case examples in the accompanying boxes in this article.
Increased positive or negative spherical aberration will refract as hyperopia and myopia, respectively, while coma refracts as astigmatism, often confusing both the power and the axis of the real astigmatic component. This must be taken into account when targeting the spherocylindrical correction in treatment of secondary cases with distorted corneal optics.
Fundamental 3: UNEXPECTED REFRACTIVE INFLUENCE OF THE POSTERIOR CORNEAL SURFACE
Total corneal power has traditionally been calculated on the basis of the anterior corneal curvature, using the keratometric refractive index of 1.3375, to compensate for the effect of the posterior surface. Neglecting the real influence of the posterior corneal surface in vision-correction planning may not result in errors if the profiles of anterior and posterior surfaces follow each other. However, if there is a discrepancy between the two, the influence of the posterior surface on the total corneal refraction can be significant,18-20 and traditional measurements based only on anterior corneal curvature may not be sufficient.
In keratoconus or irregular astigmatism secondary to incisional refractive surgery, major refractive changes originate from the posterior surface and are balanced by less pronounced changes of similar profile on the anterior surface. In such cases, regularization of the anterior surface via topography-guided ablation may break the balance and lead to manifesting the refractive errors originating from the posterior surface. This has been shown in some cases in which significantly increased ocular HOAs and lower visual performance were seen in keratoconic eyes upon correction with rigid gas-permeable lenses.21,22
The contribution of the corneal posterior surface to the eye's optical system may be significant and should be evaluated upon treatment planning; this is especially important in conditions in which significant posterior corneal optical irregularities are compensated by reciprocal changes of the anterior corneal surface.
Fundamental 4: CENTRATION AND TILT IN CUSTOM ABLATION TREATMENT OF DECENTERED CORNEAL OPTICS
Centration of the laser ablation in corneal refractive surgery is essential for achieving good visual outcomes. The literature focuses mostly on comparing centration on the corneal vertex (ie, coaxially sighted corneal light reflex), which closely approximates the corneal intercept of the visual axis,23 with centration on the entrance pupil. Using one or the other of these approaches when treating virgin eyes for regular myopia does not lead to major differences in outcomes, but the superiority of centering on the corneal vertex has been widely accepted in treating eyes with large angle kappa and hyperopia.24-27
The tilt of the axis on which the ablation plan is built is not commonly addressed in conventional ablation planning, as it does not seem to cause problems in the treatment of virgin eyes and in most simple enhancements. However, the issue arises in eyes with optical asymmetry after decentered refractive treatments or in keratoconus, as the visual axis may shift from its original (physiologic) position to a new one as the eye adapts to changed optical circumstances by assuming a new rotational position to achieve the best quality image on the fovea. This hypothesis has been demonstrated in eyes with keratoconus.28
An ablation plan using topography or aberrometry information referenced to the visual axis (or line of sight) in an eye with disturbed optical symmetry would attempt to optimize the corneal optics on the basis of the pathologic rotational position. In that case, the ablation plan would include treating significant tilt, which would usually lead to ablation of much more tissue than necessary and to an abrupt transition from treated to untreated cornea.29 The simplest way to ameliorate this issue is to identify the tilt component from the preoperative topography or wavefront on which the custom ablation is based and to exclude it from the ablation plan.
A more elaborate approach to the problem is employed by the Corneal Interactive Programmed Topographic Ablation planning software (CIPTA; iVis Technologies). iVis Technologies introduced the term restored morphologic axis to refer to an axis of corneal symmetry restored based on clues from the shape of the untouched parts of an optically decentered cornea.30
In a study investigating the influence of centration and orientation of the ablation profile on simulated outcomes of customized treatments of secondary irregular astigmatism after decentered ablations, two ablation simulations based on the visual or corneal morphologic axis were compared.29 The investigators found that treatments based on the corneal morphologic axis attempted to recenter the primary treatment by placing the ablation on the previously untreated cornea; in contrast, treatments based on the visual axis attempted to optimize the area of the decentered treatment, increasing the corneal asymmetry, using more tissue from the already treated area, and increasing the transition zone gradient toward the untreated cornea. The results showed that ablation based on the corneal morphologic axis appeared to minimize corneal tissue consumption and allowed the use of a smoother transition zone.
In cases of secondary corneal optical asymmetry, the eye will change its original rotational position during fixation, introducing a pathologic tilt to all measurements used for custom ablation planning. For good results in these eyes, it is necessary either to exclude the tilt component from the ablation plan or to use specialized solutions such as the aforementioned CIPTA software.
Case No. 1
A 38-year-old man who underwent myopic PRK (-4.50 D in each eye) developed postoperative haze in his right eye. He was treated 3 months later with use of a diamond burr. After 7 months, he was referred for topography-guided therapeutic surgery due to visual disturbances, reduced distance BCVA, and hyperopia with a manifest refraction of +8.00 -1.00 X 5˚. Irregular astigmatism and hyper-oblate asphericity, with Q-values within the central 3.5 mm of 2.41 and 3.72 for anterior and total (anterior plus posterior) cornea, respectively, were detected by Scheimpflug topography/tomography (Figure 1). Wavefront aberrometry showed increased HOAs, dominated by corneal coma and spherical aberration. Biometry measurements showed comparable axial lengths in his two eyes, and the average keratometry (K) showed that the cornea in the right eye was 3.50 D flatter than in the left.
Due to the multifocality of the oblate cornea, determining the target for spherical correction was difficult. However, we could reason that both eyes had a similar refraction before the initial refractive surgery, as well as compatible axial lengths, while the right eye refracted 8.00 D versus the left eye, which was near emmetropic. Because the average K showed only 3.50 D difference between the eyes, we opted to correct 3.50 D, assuming that the residual amount of hyperopia was due to spherical aberration refracting as positive sphere.
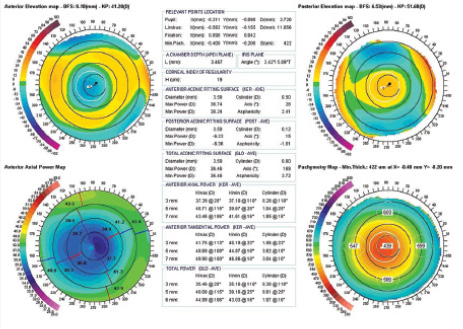
Figure 1. Scheimpflug topography/tomography (Precisio; iVis Technologies) showing (clockwise) anterior elevation, posterior elevation, axial keratometric topography, and pachymetry map in a patient with irregular astigmatism and hyper-oblate asphericity.
Fundamental 5: THE ISSUE OF ALIGNMENT
Precise overlap of successive laser pulses on the cornea in accordance with the ablation plan is a prerequisite for a well-executed treatment. With the help of an eye tracker, eye movements can be recognized and the laser beam repositioned in the x and y planes before the next laser pulse is delivered. There are two requirements for accurate laser delivery: orthogonal centration and the ability to address cyclotorsional misalignment.31 The latter requirement increases in significance with the complexity of the ablation pattern.32
Case No. 2
A 36-year-old man underwent LASIK for moderate myopia, which resulted in decentered optics in his right eye, causing visual disturbances in the form of multiplopia. At the examination before the repair surgery with topography-guided ablation, his topography showed optical asymmetry (Figure 1), classified as corneal coma by Zernicke analysis of his cornea.
Subjectively, the patient's refraction was plano -1.25 X 42˚, while corneal astigmatism as measured by topography showed cylinder of -0.58 X 131˚ that amounted to a difference of 1.83 D. The internal astigmatism as measured with OPD Scan II (Nidek) before the primary surgery was close to the posterior corneal astigmatism measured by Scheimpflug topography (-0.39 X 176˚), and this did not change after the primary surgery, indicating that there was no significant intraocular astigmatism component present. Therefore, the discrepancy between the manifest and corneal astigmatism was ascribed to coma, which was considered to have refracted as astigmatism. Consequently, topographic regularization of the cornea was targeted without taking the subjective astigmatism into account, with the expectation that correction of the coma-provoking decentered optics would result in appropriate treatment of the astigmatism.
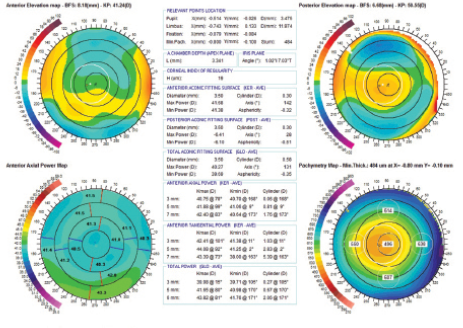
Figure 1. Precisio topography/tomography showing (clockwise) anterior elevation, posterior elevation, axial keratometric topography, and pachymetry map before repair surgery in a patient with optical asymmetry causing coma.
Ocular cyclotorsional movement may occur as a result of the patient shifting from a seated to supine position, head tilting, head rotation, autorotation, and distortion of the eye by the lid speculum.33,34 Large cyclotorsional errors can lead to significantly worse outcomes after treatment for astigmatism,35 resulting in induced astigmatism and HOAs.
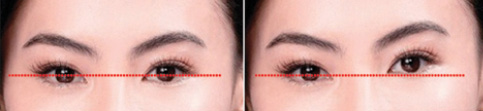
Figure 2. Schematic drawing showing a patient's head position during custom data acquisition; correct leveling, where a horizontal line intersects the pupil center of the right and left eyes (A), and incorrect leveling (B) are shown.
Several approaches have been used to address this issue, including manual limbal marking with subsequent eye and laser astigmatic axis alignment, and automated cyclotorsional tracking. Although marks can fade and manual methods are prone to human error,36 studies have shown that the manual technique reduced cyclotorsional registration error37 and improved the outcomes of astigmatic correction.38 Iris recognition technology has been implemented in cyclotorsional eye trackers that continuously scan the iris for preoperatively acquired landmarks and adjust the placement of the laser beam to match them.39
Correcting for differences in cyclotorsional eye position between the time of preoperative iris information capture and surgery, including the dynamic eye rotation occurring during the surgery, has been reported to improve the predictability of outcomes of astigmatism correction.40 However, in cases in which manifest refraction is used as the basis for the treatment of astigmatism, discrepancy between the cyclotorsional position during the subjective refraction examination (when the astigmatic axis is determined) and during iris image capture may occur (Figure 2). This discrepancy may lead to registration error, even with an otherwise perfectly functioning cyclotorsional tracking system, and this may be the reason that some studies have showed no significant difference between outcomes with and without cyclotorsional tracking41 or between automated cyclotorsional tracking and manual limbal marking.42 To prevent head-tilt discrepancies, a leveling technique can be employed, in which the height of the pupils is kept constant at all relevant examinations (Figure 2).
Iris–registration-based eye trackers detect and correct cyclotorsional errors automatically. However, if subjective refraction is used for astigmatic axis determination, caution must be taken to keep the same cyclotorsional eye position during preoperative manifest refraction examination and iris pattern acquisition. The limbal marking technique, combined with a leveling technique, is recommended when automated cyclotorsional tracking is not available or as a precautionary measure in case the cyclotorsional tracking technology fails.
CONCLUSION
The five issues discussed above may go unnoticed when treating low to moderate myopia in virgin eyes, but the more difficult the case we are treating, the more devastating errors we may make. Complex cases with irregular astigmatism secondary to complicated refractive or corneal surgery, patients with keratoconus, or eyes with optical irregularities caused by scarring after corneal injuries or keratitis should always direct our attention toward these issues. n
Case No. 3
A candidate referred for topography-guided custom ablation for irregular astigmatism was previously treated for high astigmatism by a series of RK, AK, and PRK procedures. The patient presented with a distance BCVA of 20/40 in her left eye with correction of +0.75 -2.50 X 95°. Orbscan topography (Bausch + Lomb) of the anterior and posterior corneal surfaces showed irregular astigmatism with a pronounced posterior protrusion (Figure 1).
Ablation aimed at correcting hyperopia and the anterior corneal irregular astigmatism was performed (Figure 2), and a regular anterior corneal surface was achieved (Figure 3). However, distance BCVA did not improve as expected, but was rather reduced from 20/40 to 20/63 with manifest refraction of +2.75 -2.75 X 177°. Compared with the preoperative refraction, the astigmatism was overcorrected by about 200% (from -2.50 D at the horizontal meridian to -2.75 D at the vertical meridian) as a result of the anterior corneal regularization.
This case illustrates that potentially visually significant irregularities of the posterior corneal surface occurring after incisional refractive surgery and in keratoconus are often covered (balanced) by reciprocal anterior surface irregularities. Correcting the latter will disturb that balance and degrade the total corneal optics despite an apparently improved anterior topography.
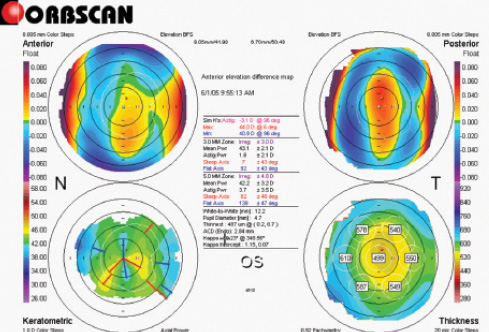
Figure 1. Orbscan topography/tomography showing (clockwise) anterior elevation, posterior elevation, axial keratometric topography, and pachymetry map before repair surgery secondary to incisional surgery in a patient with anterior and posterior protrusion along the vertical meridian.
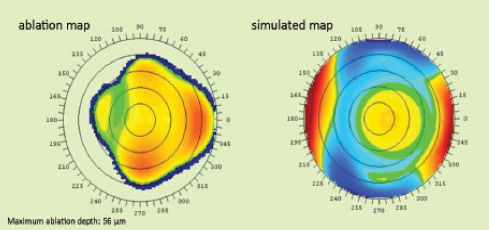
Figure 2. A topography-guided ablation map (A) and simulated postoperative anterior corneal topography (B) generated by CIPTA software.
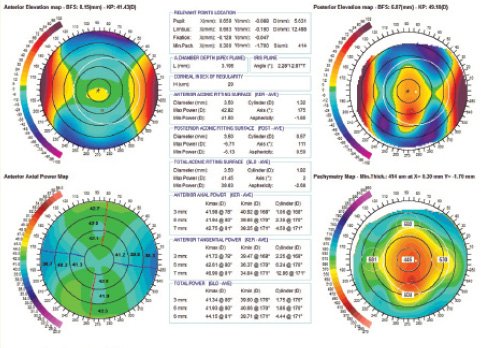
Figure 3. Precisio topography/tomography showing (clockwise) anterior elevation, posterior elevation, and pachymetry map after topography-guided ablation. Anterior elevation shows an optically regularized, symmetric profile, while posterior elevation remains irregular.
1. Simon G, Ren Q, Kervick GN, Parel JM. Optics of the corneal epithelium. Refract Corneal Surg. 1993;9(1):42-50.
2. Reinstein DZ, Archer TJ, Gobbe M, et al. Epithelial thickness in the normal cornea: three-dimensional display with Artemis very high-frequency digital ultrasound. J Refract Surg. 2008;24(6):571-581.
3. Reinstein DZ, Archer TJ, Gobbe M. Rate of change of curvature of the corneal stromal surface drives epithelial compensatory changes and remodeling. J Refract Surg. 2014;30(12):799-802.
4. Wang J, Thomas J, Cox I, Rollins A. Noncontact measurements of central corneal epithelial and flap thickness after laser in situ keratomileusis. Invest Ophthalmol Vis Sci. 2004;45(6):1812-1816.
5. Spadea L, Fasciani R, Necozione S, Balestrazzi E. Role of the corneal epithelium in refractive changes following laser in situ keratomileusis for high myopia. J Refract Surg. 2000;16(2):133-139.
6. Reinstein DZ, Srivannaboon S, Gobbe M, et al. Epithelial thickness profile changes induced by myopic LASIK as measured by Artemis very high-frequency digital ultrasound. J Refract Surg. 2009;25(5):444-450.
7. Patel SV, Erie JC, McLaren JW, Bourne WM. Confocal microscopy changes in epithelial and stromal thickness up to 7 years after LASIK and photorefractive keratectomy for myopia. J Refract Surg. 2007;23(4):385-392.
8. Gauthier CA, Holden BA, Epstein D, et al. Factors affecting epithelial hyperplasia after photorefractive keratectomy. J Cataract Refract Surg. 1997;23(7):1042-1050.
9. Reinstein DZ, Archer TJ, Dickeson ZI, Gobbe M. Transepithelial phototherapeutic keratectomy protocol for treating irregular astigmatism based on population epithelial thickness measurements by artemis very high-frequency digital ultrasound. J Refract Surg. 2014;30(6):380-387.
10. Zhou W, Stojanovic A. Comparison of corneal epithelial and stromal thickness distributions between eyes with keratoconus and healthy eyes with corneal astigmatism >/= 2.0 D. PLoS One. 2014;9(1):e85994.
11. Kanellopoulos AJ, Asimellis G. OCT corneal epithelial topographic asymmetry as a sensitive diagnostic tool for early and advancing keratoconus. Clin Ophthalmol. 2014;8:2277-2287.
12. Wu S, Tao A, Jiang H, et al. Vertical and horizontal corneal epithelial thickness profile using ultra-high resolution and long scan depth optical coherence tomography. PLoS One. 2014;9(5):e97962.
13. Reinstein DZ, Archer TJ, Gobbe M, et al. Repeatability of layered corneal pachymetry with the artemis very high-frequency digital ultrasound arc-scanner. J Refract Surg. 2010;26(9):646-659.
14. Applegate RA, Ballentine C, Gross H, et al. Visual acuity as a function of Zernike mode and level of root mean square error. Optom Vis Sci. 2003;80(2):97-105.
15. Applegate RA, Marsack JD, Thibos LN. Metrics of retinal image quality predict visual performance in eyes with 20/17 or better visual acuity. Optom Vis Sci. 2006;83(9):635-640.
16. Rouger H, Benard Y, Legras R. Effect of monochromatic induced aberrations on visual performance measured by adaptive optics technology. J Refract Surg. 2010;26(8):578-87.
17. Applegate RA, Sarver EJ, Khemsara V. Are all aberrations equal? J Refract Surg. 2002;18(5):S556-562.
18. Ho JD, Tsai CY, Liou SW. Accuracy of corneal astigmatism estimation by neglecting the posterior corneal surface measurement. Am J Ophthalmol. 2009;147(5):788-795, 95.e1-2.
19. Koch DD, Ali SF, Weikert MP, et al. Contribution of posterior corneal astigmatism to total corneal astigmatism. J Cataract Refract Surg. 2012;38(12):2080-2087.
20. Chen M, Yoon G. Posterior corneal aberrations and their compensation effects on anterior corneal aberrations in keratoconic eyes. Invest Ophthalmol Vis Sci. 2008;49(12):5645-5652.
21. Negishi K, Kumanomido T, Utsumi Y, Tsubota K. Effect of higher-order aberrations on visual function in keratoconic eyes with a rigid gas permeable contact lens. Am J Ophthalmol. 2007;144(6):924-929.
22. Marsack JD, Parker KE, Pesudovs K, et al. Uncorrected wavefront error and visual performance during RGP wear in keratoconus. Optom Vis Sci. 2007;84(6):463-470.
23. Pande M, Hillman JS. Optical zone centration in keratorefractive surgery. Entrance pupil center, visual axis, coaxially sighted corneal reflex, or geometric corneal center? Ophthalmology. 1993;100(8):1230-7.
24. Okamoto S, Kimura K, Funakura M, et al. Comparison of wavefront-guided aspheric laser in situ keratomileusis for myopia: coaxially sighted corneal-light-reflex versus line-of-sight centration. J Cataract Refract Surg. 2011;37(11):1951-60.
25. Okamoto S, Kimura K, Funakura M, et al. Comparison of myopic LASIK centered on the coaxially sighted corneal light reflex or line of sight. J Refract Surg. 2009;25(10 Suppl):S944-50.
26. Reinstein DZ, Archer TJ, Gobbe M. Is topography-guided ablation profile centered on the corneal vertex better than wavefront-guided ablation profile centered on the entrance pupil? J Refract Surg. 2012;28(2):139-143.
27. Reinstein DZ, Gobbe M, Archer TJ. Coaxially sighted corneal light reflex versus entrance pupil center centration of moderate to high hyperopic corneal ablations in eyes with small and large angle kappa. J Refract Surg. 2013;29(8):518-525.
28. Mihaltz K, Kranitz K, Kovacs I, et al. Shifting of the line of sight in keratoconus measured by a hartmann-shack sensor. Ophthalmology. 2010;117(1):41-8.
29. Stojanovic A, Suput D. Strategic planning in topography-guided ablation of irregular astigmatism after laser refractive surgery. J Refract Surg. 2005;21(4):369-376.
30. Alessio G LTM, Sborgia C. Corneal interactive programmed topographic ablation using the lasersight laser. In: MacRae SM KR, Applegate RA, eds. Customized Corneal Ablation. Thorofare, NJ: Slack Inc, 2001.
31. Waring GO 3rd. One-kilohertz eye tracker and active intraoperative torsion detection in the NIDEK CXIII and Quest excimer lasers. J Refract Surg. 2009;25(10 Suppl):S931-933.
32. Bueeler M, Mrochen M, Seiler T. Maximum permissible torsional misalignment in aberration-sensing and wavefront-guided corneal ablation. J Cataract Refract Surg. 2004;30(1):17-25.
33. Suzuki A, Maeda N, Watanabe H, et al. Using a reference point and videokeratography for intraoperative identification of astigmatism axis. J Cataract Refract Surg. 1997;23(10):1491-1495.
34. Ciccio AE, Durrie DS, Stahl JE, Schwendeman F. Ocular cyclotorsion during customized laser ablation. J Refract Surg. 2005;21(6):S772-4.
35. Tjon-Fo-Sang MJ, de Faber JT, Kingma C, Beekhuis WH. Cyclotorsion: a possible cause of residual astigmatism in refractive surgery. J Cataract Refract Surg. 2002;28(4):599-602.
36. Igarashi A, Kamiya K, Shimizu K. Clinical evaluation of accuracy of horizontal meridian limbal marking. Optom Vis Sci. 2013;90(6):540-545.
37. Chen X, Stojanovic A, Stojanovic F, et al. Effect of limbal marking prior to laser ablation on the magnitude of cyclotorsional error. J Refract Surg. 2012;28(5):358-362.
38. Farah SG, Olafsson E, Gwynn DG, et al. Outcome of corneal and laser astigmatic axis alignment in photoastigmatic refractive keratectomy. J Cataract Refract Surg. 2000;26(12):1722-1728.
39. Chernyak DA. Iris-based cyclotorsional image alignment method for wavefront registration. IEEE Trans Biomed Eng. 2005;52(12):2032-2040.
40. Venter J. Outcomes of myopic LASIK with and without NIDEK active torsion error correction. J Refract Surg. 2009;25(11):985-990.
41. Moshirfar M, Chen MC, Espandar L, et al. Effect of iris registration on outcomes of LASIK for myopia with the VISX CustomVue platform. J Refract Surg. 2009;25(6):493-502.
42. Shen EP, Chen WL, Hu FR. Manual limbal markings versus iris-registration software for correction of myopic astigmatism by laser in situ keratomileusis. J Cataract Refract Surg. 2010;36(3):431-436.
Aleksandar Stojanovic, MD
- Senior Consultant in charge of refractive surgery and keratoconus, Eye Department, University Hospital North, Norway
- Medical Director, SynsLaser Clinic, Tromsoe and Oslo, Norway
- aleks@online.no
- Financial disclosure: None
Wen Zhou, MD
- Researcher at SynsLaser Clinic, Tromsoe and Oslo, Norway
- mosquito.0907@me.com
- Financial disclosure: None