CXL using ultraviolet-A (UV-A) light and riboflavin was developed in the 1990s to treat corneal ectatic disorders, such as keratoconus and post-LASIK and post-PRK iatrogenic corneal ectasias. CXL soon became the gold standard in multiple countries around the world to halt the progression of these disorders, with good long-term (>10 years) outcomes in keratometry readings and visual acuity.1 By introducing the procedure in our respective countries, Italy (C.M.) and India (S.J.), we have contributed to the ongoing evolution of CXL by developing two original techniques—epithelial island CXL (EI-CXL)2 and contact lens–assisted CXL (CA-CXL)3—for the treatment of ectatic corneas (Figure 1).
AT A GLANCE
•Conventional and accelerated CXL protocols have demonstrated medium- to long-term improvement in visual acuity and topographic and aberrometric parameters; however, the results are often variable and unpredictable due to the uneven biologic response of collagen to photodynamic reaction.
• Early diagnosis of corneal ectasia is mandatory, especially in pediatric patients who generally demonstrate faster progression.
• Today, the CXL therapeutic window allows treatment in about 20 minutes, with a good balance between efficacy and tolerability for the patient, optimizing the time of surgery without affecting treatment efficacy.
Using in vivo scanning laser confocal microscopy (IVCM), we demonstrated for the first time that, although conventional CXL efficiently strengthened the cornea, it killed most of the keratocytes within the anterior to mid-corneal stroma; these cells later repopulated without affecting the corneal endothelium, the lens, or the retina.4
.jpg)
Figure 1. Dr. Mazzotta’s epithelial island CXL (A) and Dr. Jacob’s contact lens–assisted CXL (B) techniques for thin corneas.
Conventional epithelium-off (epi-off) CXL has been shown to be safe and effective over time and to stabilize progressive keratoconus and secondary ectasia in multiple clinical trials.1,4 The procedure is time-consuming, however (taking about 1 hour), and it leads to consequent patient discomfort. A CXL procedure that addresses these drawbacks would be highly desirable.
ACCELERATED PROTOCOLS
The basic physical concept stated in the Bunsen-Roscoe law of reciprocity supports the performance of new and evolving accelerated CXL protocols according to the so-called equal-dose principle. By setting the UV-A power at 9 mW/cm2 for 10 minutes, 30 mW/cm2 for 3 minutes, 18 mW/cm2 for 5 minutes, or 45 mW/cm2 for 2 minutes, while maintaining a constant energy dose of 5.4 J/cm2 delivered to the corneal tissue, we can achieve the same effect as the standard Dresden protocol at 3 mW/cm2 for 30 minutes.
Using IVCM analysis to investigate different accelerated CXL protocols with continuous and fractionated high-irradiance UV-A light exposure, we recently showed significant differences in keratocyte apoptosis (ie, treatment penetration).4 These differences may have serious biomechanical and clinical implications in the long-term stabilization of ectasia and in the functional impact of CXL treatments.
The 9 mW/cm2 accelerated CXL was safe for corneal endothelium, stabilizing the progression of keratoconus and iatrogenic ectasia with significant reduction in topographic keratometric values and significant increase in distance BCVA that was comparable with conventional 3 mW/cm2 CXL at midterm (24 months) follow-up.5,6 Randomized controlled long-term studies are, however, required to confirm the encouraging results and noninferiority to conventional treatment in the long term.
In a recent study,7 accelerated CXL with 18 mW/cm2 UV-A power showed less topographic flattening compared with conventional CXL. Refractive and visual results of the accelerated CXL method using continuous light and pulsed-light treatment at 30 mW/cm2 and 7.2 J/cm2 energy dose gave similar results compared to conventional 3 mW/cm2 CXL after 12 months of follow-up.8,9 The accelerated CXL method provided a faster recovery and greater patient comfort; however, the depth of the demarcation line after 30 mW/cm2 was less than 200 μm of corneal stroma, which may be the limit of this technique.
IVCM and corneal OCT data verified the presence of postoperative cell apoptosis, nerve disappearance, and demarcation lines induced by accelerated CXL. These imaging modalities also documented a mean treatment penetration of 150 µm (range, 140 µm to 200 µm, measured from the epithelial surface) after 30 mW/cm2 continuous-light accelerated CXL. By contrast, penetration was 220 µm (range, 200 µm to 250 µm, measured from the epithelial surface) after fractionated UV-A light accelerated CXL. In transepithelial standard and accelerated CXL, limited and uneven cell apoptosis was confined to the anterior stroma under Bowman layer at a mean depth of 80 µm (range, 50 µm to 100 µm), with no evidence of demarcation lines on IVCM or spectral-domain corneal OCT (Figures 2 and 3).
Moreover, limited biomechanical transfer was documented by Brillouin microscopy testing,10 and insufficient (short-term) ectasia stabilization was shown in 2-year follow-up clinical studies, especially in patients aged 18 years and younger with faster keratoconus progression.11
Based on existing evidence of CXL’s safety and efficacy, in September 2013 the National Institute for Health and Care Excellence in the United Kingdom recommended epi-off CXL as a benchmark for conservative treatment of progressive ectasia.12
FRACTIONATED LIGHT ACCELERATED CXL
Our studies of accelerated CXL showed that increasing UV-A power while reducing treatment exposure times achieved limited penetration. This was also demonstrated after continuous-light accelerated CXL with 30 mW/cm2. On the other hand, fractionating UV-A light exposure yielded better penetration, as shown on IVCM analysis.8,9 Deeper cell viability is achievable by prolonging the exposure time while maintaining the same energy dose delivered to the corneal tissue.
.jpg)
Figure 2. Demarcation line on IVCM (A) and on spectraldomain corneal OCT (B) after high-irradiance fractionated light (1 second on/1 second off) 30 mW accelerated CXL.
One-year functional results of epi-off pulsed-light accelerated CXL demonstrated keratoconus stability with good functional outcomes and deeper stromal penetration.8 No endothelial damage was seen during follow-up after accelerated CXL.
Our studies support earlier suggestions that oxygen represents the main driver of the collagen-CXL reaction; it may be that pulsed-light treatment may optimize the intraoperative availability of oxygen in CXL, improving postoperative functional outcomes compared with continuous-light treatments.
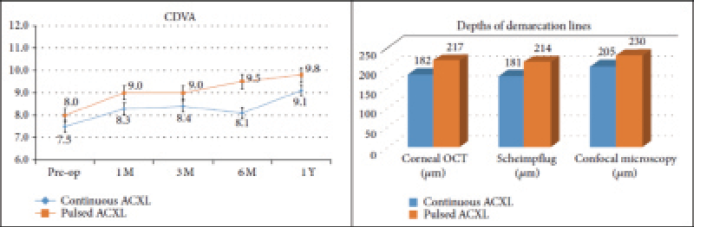
Figure 3. Functional visual acuity results (left) and demarcation line depth (right) after high-irradiance continuous and fractionated light exposure (1 second on/1 second off)30 mW accelerated CXL.
.jpg)
Figure 4. Demarcation lines with different accelerated CXL protocols. Penetration of 9 mW/cm2 contact lensassisted CXL at 357 μm with epithelium (A). Demarcation line of 30 mW/cm2 pulsed-light CXL reaching approximately 180 μm stromal penetration with epithelium (B). Demarcation line of 15 mW/cm2 contact lens-assisted CXL with pulsed light, at 282 μm (C). Each protocol was performed at the Siena International Crosslinking Center by Dr. Mazzotta using the standard energy dose of 5.4 J/cm2.
In our opinion, to ensure a long-lasting stability of keratoconus and secondary ectasia, UV-A power setting and exposure time should be targeted to allow a treatment penetration at least at 250 μm, overcoming the so-called stiff cornea. To achieve this goal, while maintaining the standard 5.4 J/cm2 dose delivery, the UV-A power can be calibrated between 9 mW/cm2 and 15 mW/cm2 with pulsed light. These data have been preliminarily tested in a laboratory study13 demonstrating that effective crosslinking requires the presence of oxygen in addition to sufficient penetration of riboflavin and U-VA exposure. This study showed that high-irradiance (accelerated) CXL was equally efficacious as the conventional protocol, and better strain (elongation) test results were achieved with 15 mW/cm2 at 5.4 J/cm2 with continuous (6 minutes) or fractionated (30 seconds on/30 seconds off) U-VA exposure.
At the Siena International Crosslinking Center, we now have 24 months of clinical experience with the 15 mW/cm2 accelerated CXL protocol with 10 minutes fractionated light and 5.4 J/cm2 energy dose. Our results compare well with the laboratory data discussed above, demonstrating that demarcation lines are at a depth of between 280 μm and 320 μm on average (±30 μm standard deviation) with no endothelial damage. Functional data are comparable with those of conventional CXL with 24 months’ follow-up (Figure 4).
TOPOGRAPHY-GUIDED CXL
In order to improve the quality of vision of patients with keratoconus while maintaining stability, we are evolving topography-guided accelerated CXL protocols with a customized energy dose release according to corneal curvature (Figure 5). These protocols are based on patient-specific computational modeling of keratoconus progression and differential responses to CXL. According to computational analysis,14 smaller-diameter simulated CXL treatments centered on the cone provided greater reductions in corneal curvature and higher-order aberrations than conventional broad-beam CXL patterns.
When doses of energy higher than the standard 5.4 J/cm2 (ie, 7.2 J/cm2, 10 J/cm2, and 15 J/cm2) were used, keratocyte apoptosis was detected between the depth of 250 µm and 300 µm. IVCM and corneal OCT scans revealed multiple demarcation lines underlying different depths corresponding to the energy doses used according to the topography-guided zonal CXL principle.15
A NEW HYPOTHESIS
These preliminary observations allowed us to formulate a new hypothesis regarding the understanding of CXL-induced biodynamic interactions: CXL treatment volume is related not only to exposure time and UV-A power but also to energy dose. According to our IVCM analysis, using the conventional energy dose of 5.4 J/cm2, the stromal penetration of CXL (as marked by cell apoptosis) appeared to be inversely proportional to UV-A power—the lower the UV-A power, the deeper the penetration, and the higher the UV-A power, the lower the penetration—and directly proportional to exposure time—the shorter the time, the lower the penetration, and the longer the time, the deeper the penetration. The same basic concepts were applicable to the 7.2 J/cm2 energy dose.
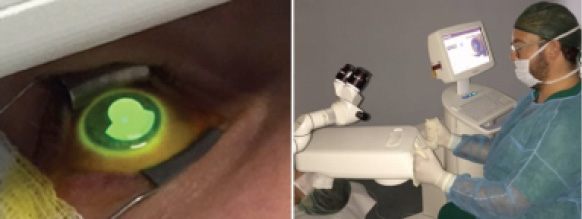
Figure 5. Topography-guided accelerated CXL treatment pattern (A) and Avedro’s KXL II CXL UV-A source for topography-guided accelerated CXL (B).
After high-irradiance, fractionated accelerated CXL with 7.2 J/cm2, our IVCM data showed slightly more hyper-reflective stromal tissue, which might be correlated with higher collagen compaction compared with the 5.4 J/cm2 energy dose. No dose-dependent substantial differences were found between these two experimental settings. IVCM results suggested that, by using an energy dose greater than 7.2 J/cm2, we could achieve higher penetration along with reduced exposure time and increased UV-A power. This research may change the application of CXL techniques and customization of protocols.
Other important variables influencing postoperative cell apoptosis are riboflavin concentration and soaking time. Recently, the advent of dextran-free 0.1% riboflavin formulas has provided a compromise between the necessity to maintain optimal treatment penetration with faster soaking time and the safety of endothelial cells provided by the riboflavin shield and hydroxypropyl methylcellulose vehicles.
SUMMARY
Conventional and accelerated CXL protocols have demonstrated medium- to long-term improvement in visual acuity and topographic and aberrometric parameters; however, the results are often variable and unpredictable due to the uneven biologic response of collagen to photodynamic reaction. Epi-off CXL represents an optimal solution for progressive early ectasia, in which our aim is to freeze the disease indefinitely in patients who generally have good BCVA and few higher-order aberrations, preventing them from needing corneal transplants.
CXL is not a refractive procedure, and the primary goal of this therapy is to stabilize corneal ectasia. Early diagnosis is mandatory, especially in pediatric patients who generally demonstrate faster progression. Greater dissemination of information and awareness about the health risks associated with keratoconus, such as the associated visual deterioration and the potential need for corneal transplantation, is strongly recommended. This information should be specifically geared toward specialists and children.
Today, the CXL therapeutic window allows treatment in about 20 minutes in all cases with a good balance between efficacy and tolerability for the patient, optimizing the time of surgery without affecting treatment efficacy. The CXL treatment of the future will be customized, and greater attention will be given to obtaining a refractive effect through focal treatment enhanced to increase the flattening of the most ectatic areas while inducing a compensatory steepening of the flattest. Treatment protocols guided by topography are evolving to achieve a nonablative and nonincisional refractive response without sacrificing the stabilizing effect of the original crosslinking concept.
1. Raiskup F, Theuring A, Pillunat LE, Spoerl E. Corneal collagen crosslinking with riboflavin and ultraviolet-A light in progressive keratoconus: ten-year results. J Cataract Refract Surg. 2015;41(1):41-46.
2. Mazzotta C, Ramovecchi V. Customized epithelial debridement for thin ectatic corneas undergoing corneal cross-linking: epithelial island cross-linking technique. Clin Ophthalmol. 2014;8:1337-1343.
3. Jacob S, Kumar DA, Agarwal A, Basu S, Sinha P, Agarwal A. Contact lens-assisted collagen cross-linking (CACXL): a new technique for cross-linking thin corneas. J Refract Surg. 2014;30(6):366-372.
4. Mazzotta C, Hafezi F, Kymionis G, et al. In vivo confocal microscopy after corneal collagen crosslinking. Ocul Surf. 2015;13(4):298-314.
5. Elbaz U, Shen C, Lichtinger A, et al. Accelerated (9-mW/cm2) corneal collagen crosslinking for keratoconus—a 1-year follow-up. Cornea. 2014;33(8):769-773.
6. Marino GK, Torricelli AA, Giacomin N, Santhiago MR, Espindola R, Netto MV. Accelerated corneal collagen cross-linking for postoperative LASIK ectasia: two-year outcomes. J Refract Surg. 2015;31(6):380-384.
7. Chow VW, Chan TC, Yu M, Wong VW, Jhanji V. One-year outcomes of conventional and accelerated collagen crosslinking in progressive keratoconus. Sci Rep. 2015;5:14425.
8. Mazzotta C, Traversi C, Paradiso AL, Latronico ME, Rechichi M. Pulsed light accelerated crosslinking versus continuous light accelerated crosslinking: one-year results. J Ophthalmol. 2014;2014:e604731.
9. Mazzotta C, Traversi C, Caragiuli S, Rechichi M. Pulsed vs continuous light accelerated corneal collagen crosslinking: in vivo qualitative investigation by confocal microscopy and corneal OCT. Eye (Lond). 2014;28(10):1179-1183.
10. Scarcelli G, Kling S, Quijano E, Pineda R, Marcos S, Yun SH. Brillouin microscopy of collagen crosslinking: noncontact depth-dependent analysis of corneal elastic modulus. Invest Ophthalmol Vis Sci. 2013;54(2):1418-1425.
11. Caporossi A, Mazzotta C, Paradiso AL, Baiocchi S, Marigliani D, Caporossi T. Transepithelial corneal collagen crosslinking for progressive keratoconus: 24-month clinical results. J Cataract Refract Surg. 2013;39(8):1157-1163.
12. National Institute for Health Care and Excellence. Photochemical corneal collagen cross-linkage using riboflavin and ultraviolet A for keratoconus and keratectasia. NICE interventional procedure guidance [IPG466]. Published September 2013. Available at: http://www.nice.org.uk/guidance/ipg466. Accessed December 3, 2015.
13. Krueger RR, Herekar S, Spoerl E. First proposed efficacy study of high versus standard irradiance and fractionated riboflavin/ultraviolet a cross-linking with equivalent energy exposure. Eye Contact Lens. 2014;40(6):353-357.
14. Roy AS, Dupps WJ Jr. Patient-specific computational modeling of keratoconus progression and differential responses to collagen cross-linking. Invest Ophthalmol Vis Sci. 2011;52(12):9174-9187.
15. Mazzotta C. Data from the 11th International Crosslinking Conference. December 4-5, 2015; Boston, MA.
Cosimo Mazzotta, MD, PhD
• Professor, Ophthalmology Postgraduate Speciality School, University of Siena, Italy
• Director, Siena International Crosslinking Center
• cgmazzotta@libero.it
• Financial disclosure: None
Soosan Jacob, MS, FRCS, DNB
• Director and Chief, Dr. Agarwal’s Refractive and Cornea Foundation, Dr. Agarwal’s Group of Eye Hospitals, Chennai, India
• Member, CRST Europe Editorial Board
• dr_soosanj@hotmail.com
•Financial disclosure: None